Nutrition is a fundamental process that all living organisms must perform to sustain life. It involves the intake of nutrients from the environment, their conversion into energy, and their utilization for the growth, repair, and maintenance of cellular functions. The ways in which organisms obtain their nutrients vary widely and are broadly classified into two primary categories: autotrophic nutrition and heterotrophic nutrition.
Table of Contents
What Are Nutrients?
Nutrients are chemical substances that provide energy and are essential for the synthesis of biomolecules necessary for the physiological functions of organisms. They play a crucial role in the growth, development, and maintenance of health. Nutrients are categorized into macronutrients (carbohydrates, proteins, and fats) and micronutrients (vitamins and minerals). All living organisms require nutrients for proper functioning and development. However, the mode by which different organisms obtain these nutrients varies significantly. Some organisms can synthesize their own food using simple inorganic compounds, while others must consume complex organic compounds.
Autotrophic Nutrition: The Basics
The term autotrophic is derived from two Greek words: “autos,” meaning self, and “trophe,” meaning nutrition. Autotrophic nutrition refers to the process by which organisms synthesize their own organic food from inorganic substances available in their environment. These organisms, known as autotrophs, use either light energy or chemical energy to produce food, which is essential not only for their own survival but also for the survival of other organisms within the ecosystem.
Autotrophs are often referred to as producers because they form the base of the food chain by producing organic compounds that serve as food for other organisms, known as consumers. Autotrophic nutrition is primarily observed in plants, algae, and certain types of bacteria.
Types of Autotrophic Nutrition
Autotrophic organisms are categorized into two main types based on the source of energy they utilize for the synthesis of food:
- Photosynthetic Autotrophs (Photoautotrophs)
- Chemosynthetic Autotrophs (Chemoautotrophs)
Photosynthetic Autotrophs (Photoautotrophs)
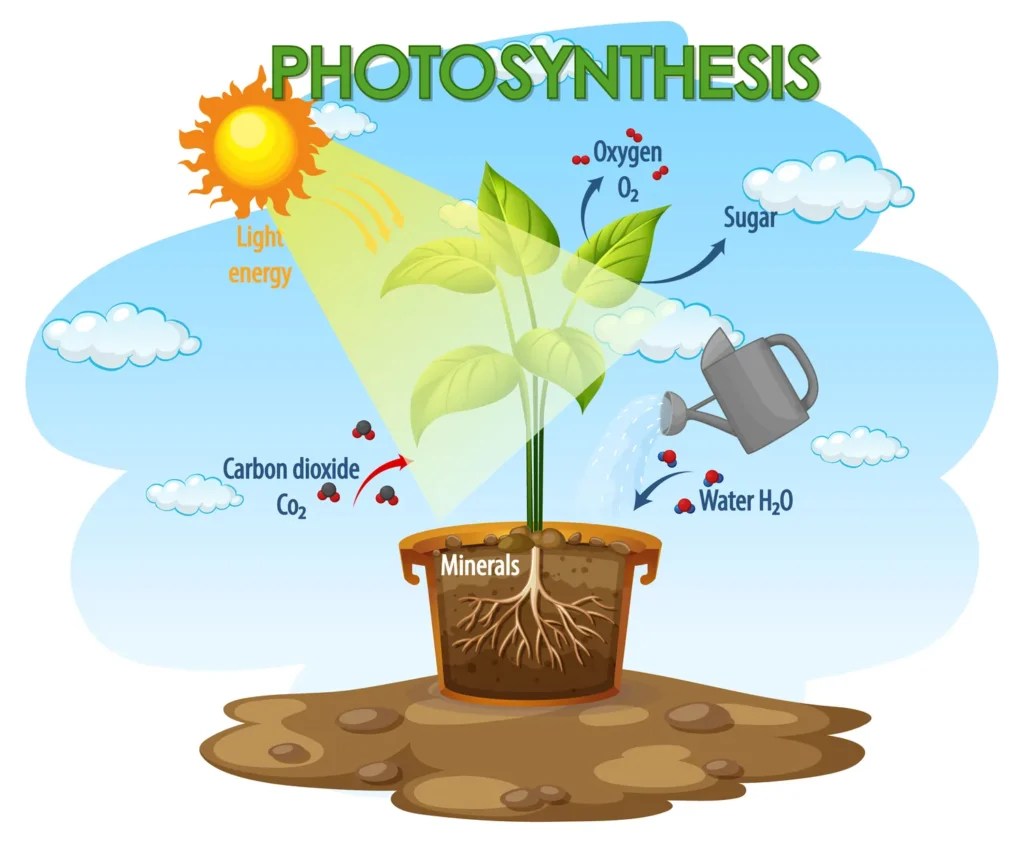
Photosynthesis is the process by which photoautotrophs convert light energy from the sun into chemical energy stored in the form of glucose, a type of sugar. This process occurs in the chloroplasts of plant cells, where the pigment chlorophyll captures light energy. The captured energy is then used to drive a series of reactions that convert carbon dioxide (CO2) and water (H2O) into glucose (C6H12O6) and oxygen (O2). The overall chemical equation for photosynthesis is as follows:
6CO2 + 6H2O + Light Energy ⟶ C6H12O6 + 6O2
This process not only produces glucose, which serves as an energy source for the plant but also releases oxygen as a byproduct, which is essential for the respiration of most other organisms on Earth.
Examples of Photosynthetic Autotrophs
- Green Plants: All green plants, from towering trees to tiny shrubs, perform photosynthesis. Examples include the mango tree (Mangifera indica), oak tree (Quercus robur), and rose bush (Rosa spp.).
- Green Algae: These are simple, aquatic plants that are capable of photosynthesis. Examples include Chlamydomonas, Volvox, and Spirogyra.
- Cyanobacteria: Also known as blue-green algae, these are a group of photosynthetic bacteria that are important in aquatic ecosystems. Examples include Anabaena and Nostoc.
Photosynthesis is crucial not only for the autotrophs themselves but also for the entire biosphere. It is the primary means by which energy from the sun is captured and made available to all other living organisms.
Chemosynthetic Autotrophs (Chemoautotrophs)
Chemosynthesis is the process by which certain autotrophs, known as chemoautotrophs, produce organic compounds using energy derived from the oxidation of inorganic molecules, rather than from sunlight. This process is particularly important in environments where sunlight is not available, such as deep-sea hydrothermal vents or underground habitats.
Chemoautotrophs play a vital role in the recycling of nutrients like nitrogen, sulfur, and iron, making them available for use by other organisms. These bacteria and archaea can be further classified based on the type of inorganic substance they oxidize:
Sub-types of Chemosynthetic Autotrophs
- Nitrifying Bacteria:
- Nitrosomonas and Nitrococcus: These bacteria oxidize ammonia (NH3) to nitrite (NO2−) in the first step of nitrification.
- Nitrobacter and Nitrocystis: These bacteria oxidize nitrite (NO2−) to nitrate (NO3−) in the second step of nitrification.
- Iron Bacteria:
- Ferrobacillus: These bacteria oxidize ferrous iron (Fe2+) to ferric iron (Fe3+), playing a role in the biogeochemical cycling of iron.
- Hydrogen Bacteria:
- Hydrogenomonas: These bacteria oxidize hydrogen gas (H2) to water (H2O), deriving energy from this reaction.
- Methane Bacteria:
- Methanomonas: These bacteria oxidize methane (CH4) to carbon dioxide (CO2), contributing to the carbon cycle.
- Sulfur Bacteria:
- Beggiatoa: These bacteria oxidize hydrogen sulfide (H2S) to elemental sulfur (S), which can be further oxidized to sulfuric acid (H2SO4) by bacteria such as Thiobacillus and Thiooxidans.
Examples of Chemosynthetic Ecosystems
- Hydrothermal Vents: Found on the ocean floor, these ecosystems are fueled by chemoautotrophic bacteria that oxidize sulfur compounds emitted from the vents. These bacteria form the base of the food chain, supporting a diverse community of organisms, including giant tube worms, clams, and shrimp.
- Sulfur Springs: In these environments, sulfur-oxidizing bacteria thrive, contributing to the unique chemistry and biology of these habitats.
Heterotrophic Nutrition: A Contrast
In contrast to autotrophic organisms, heterotrophic organisms cannot synthesize their own organic food and must rely on consuming other organisms, either autotrophs or other heterotrophs, to meet their nutritional needs. This mode of nutrition is known as heterotrophic nutrition.
Heterotrophs are classified based on their feeding habits:
- Herbivores: Organisms that feed directly on producers (e.g., cows, deer, and caterpillars).
- Carnivores: Organisms that feed on other animals (e.g., lions, wolves, and hawks).
- Omnivores: Organisms that consume both plants and animals (e.g., humans, bears, and pigs).
- Detritivores: Organisms that feed on dead organic matter (e.g., earthworms and woodlice).
- Parasites: Organisms that derive nutrients by living in or on a host organism, often causing harm (e.g., tapeworms, ticks, and mistletoe).
Importance of Autotrophic Nutrition in Ecosystems
Autotrophic nutrition is foundational to the flow of energy through ecosystems. Photoautotrophs capture solar energy and convert it into chemical energy through photosynthesis, forming the primary source of energy for almost all life forms on Earth. Without autotrophs, heterotrophic organisms would not have a consistent source of food, and the energy flow within ecosystems would collapse.
In addition, chemoautotrophs play a critical role in ecosystems that are not dependent on sunlight. By harnessing chemical energy, these organisms support unique communities in extreme environments, such as deep-sea hydrothermal vents, where sunlight does not penetrate.
Difference Between Photosynthetic Autotrophs and Chemosynthetic Autotrophs
To better understand the different modes of autotrophic nutrition and their significance, the following table summarizes key aspects, including the types of autotrophs, their energy sources, processes involved, and examples. This table provides a clear comparison between photosynthetic and chemosynthetic autotrophs, highlighting their roles in various ecosystems.
Aspect | Photosynthetic Autotrophs | Chemosynthetic Autotrophs |
---|---|---|
Definition | Organisms that synthesize organic compounds using light energy. | Organisms that synthesize organic compounds using chemical energy. |
Energy Source | Light energy (from the sun) | Chemical energy (from oxidation of inorganic compounds) |
Primary Process | Photosynthesis | Chemosynthesis |
Key Reactants | Carbon dioxide (CO₂) and water (H₂O) | Various inorganic compounds (e.g., NH₃, H₂S, CH₄, Fe²⁺) |
Products | Glucose (C₆H₁₂O₆) and oxygen (O₂) | Organic compounds (e.g., carbohydrates) and by-products (e.g., NO₃⁻, SO₄²⁻) |
Chlorophyll Presence | Yes (in chloroplasts) | No |
Examples | Green plants, green algae, cyanobacteria | Nitrifying bacteria, sulfur bacteria, iron bacteria |
Ecological Role | Primary producers in ecosystems, essential for oxygen production and energy flow. | Recyclers of nutrients in extreme environments (e.g., hydrothermal vents) |
Environment | Terrestrial and aquatic environments with sunlight | Environments without sunlight (e.g., deep-sea vents, sulfur springs) |
Importance | Supports life by providing energy and oxygen to heterotrophs. | Maintains ecosystems in extreme environments and contributes to nutrient cycling. |
Conclusion
Autotrophic nutrition is a remarkable adaptation that allows certain organisms to thrive by synthesizing their own food from inorganic substances. Whether through photosynthesis or chemosynthesis, autotrophs are essential to the balance and sustainability of ecosystems. By understanding the complexities of autotrophic nutrition, we gain insight into the fundamental processes that sustain life on Earth and the intricate relationships between producers and consumers within the web of life.
Frequently Asked Questions (FAQs) on Autotrophic Nutrition
What is autotrophic nutrition, and why is it important?
Autotrophic nutrition is the process by which certain organisms, known as autotrophs, synthesize their own organic food using simple inorganic substances available in their environment. This is accomplished either through the process of photosynthesis or chemosynthesis. Autotrophs are considered the primary producers in ecosystems because they form the base of the food chain. They convert energy from the sun (in the case of photoautotrophs) or chemical compounds (in the case of chemoautotrophs) into organic molecules like glucose, which are essential for the survival of other organisms known as heterotrophs.
The importance of autotrophic nutrition lies in its role in sustaining life on Earth by providing a continuous supply of energy and organic matter necessary for the growth, reproduction, and survival of various life forms.
Autotrophic nutrition is also crucial for the oxygenation of the Earth’s atmosphere. Through photosynthesis, photoautotrophs release oxygen as a byproduct, which is vital for the respiration of aerobic organisms, including humans. Additionally, chemoautotrophs play an essential role in nutrient cycling in ecosystems where sunlight is not available, such as deep-sea hydrothermal vents. Without autotrophic organisms, life as we know it would not be possible, as there would be no primary source of energy to fuel the biosphere.
How do photosynthetic autotrophs produce energy, and what are some examples?
Photosynthetic autotrophs produce energy through the process of photosynthesis, where they convert light energy from the sun into chemical energy stored in the form of glucose. This process occurs in the chloroplasts of plant cells, where the pigment chlorophyll absorbs light energy. The light energy drives a series of chemical reactions that convert carbon dioxide (CO₂) and water (H₂O) into glucose (C₆H₁₂O₆), which serves as an energy source for the plant. Oxygen (O₂) is released as a byproduct of this process. The overall chemical equation for photosynthesis can be represented as:
6CO2 + 6H2O + Light Energy ⟶ C6H12O6 + 6O2
Examples of photosynthetic autotrophs include green plants, such as the oak tree (Quercus robur), mango tree (Mangifera indica), and rose bush (Rosa spp.). Green algae, such as Spirogyra and Volvox, are also photosynthetic autotrophs found in aquatic environments. Additionally, cyanobacteria, also known as blue-green algae, are photosynthetic bacteria that play a crucial role in marine and freshwater ecosystems. These organisms not only produce food for themselves but also provide energy and oxygen to support a wide variety of life forms, making them indispensable to Earth’s ecosystems.
What are chemoautotrophs, and how do they differ from photoautotrophs?
Chemoautotrophs are a type of autotroph that synthesize organic compounds using chemical energy derived from the oxidation of inorganic substances, rather than light energy. This process is known as chemosynthesis. Chemoautotrophs are primarily found in environments where sunlight is absent, such as deep-sea hydrothermal vents, underground caves, and sulfur springs. They play a vital role in recycling nutrients like nitrogen, sulfur, and iron, making them available to other organisms in these extreme environments.
In contrast, photoautotrophs utilize light energy from the sun to drive the process of photosynthesis, producing organic compounds and oxygen as a byproduct. Photoautotrophs are typically found in environments with access to sunlight, such as terrestrial ecosystems and shallow aquatic habitats.
The key difference between the two lies in their energy sources: photoautotrophs rely on sunlight, while chemoautotrophs rely on the oxidation of chemical compounds. Examples of chemoautotrophs include nitrifying bacteria like Nitrosomonas and Nitrobacter, sulfur bacteria like Beggiatoa and Thiobacillus, and iron bacteria like Ferrobacillus. These organisms are crucial for maintaining the balance of ecosystems in environments where sunlight is not a viable energy source.
Can you explain the process of nitrification and its importance in the nitrogen cycle?
Nitrification is a crucial biological process in the nitrogen cycle, where specific chemoautotrophic bacteria convert ammonia (NH₃) into nitrate (NO₃⁻) through a two-step process. This process is vital for the availability of nitrogen in a form that can be readily absorbed and utilized by plants.
- First Step (Ammonia Oxidation): The first step of nitrification involves the conversion of ammonia (NH₃) to nitrite (NO₂⁻). This reaction is carried out by nitrifying bacteria such as Nitrosomonas and Nitrococcus. The reaction can be represented as
2NH3 + 3O2 ⟶ 2NO2– + 2H2O + 2H+ - Second Step (Nitrite Oxidation): In the second step, nitrite (NO₂⁻) is further oxidized to nitrate (NO₃⁻) by bacteria such as Nitrobacter and Nitrocystis. The reaction can be represented as
2NO2– + O2 ⟶ 2NO3–
Nitrate (NO₃⁻), the end product of nitrification, is a form of nitrogen that can be easily absorbed by plant roots from the soil. Plants use nitrate to synthesize essential biomolecules like amino acids, proteins, and nucleic acids. The process of nitrification is vital for maintaining soil fertility and supporting plant growth, which in turn supports the entire food chain.
Nitrification also plays a significant role in the nitrogen cycle, which is the continuous movement of nitrogen through the atmosphere, soil, water, and living organisms. By converting ammonia into nitrate, nitrifying bacteria help regulate nitrogen levels in ecosystems, preventing the accumulation of toxic ammonia and ensuring a steady supply of nitrogen for plants and other organisms.
How do autotrophs contribute to the oxygenation of Earth’s atmosphere?
Autotrophs, particularly photoautotrophs, play a critical role in the oxygenation of Earth’s atmosphere through the process of photosynthesis. During photosynthesis, photoautotrophs convert carbon dioxide (CO₂) and water (H₂O) into glucose (C₆H₁₂O₆) and oxygen (O₂) using light energy. Oxygen is released as a byproduct of this process. The overall equation for photosynthesis is:
6CO2 + 6H2O + Light Energy ⟶ C6H12O6 + 6O2
This release of oxygen by photoautotrophs, such as green plants, algae, and cyanobacteria, is the primary source of the oxygen that is present in Earth’s atmosphere. Approximately 2.4 billion years ago, during the Great Oxygenation Event, the activity of early cyanobacteria led to a significant increase in atmospheric oxygen, transforming the Earth’s atmosphere and enabling the evolution of aerobic (oxygen-dependent) life forms.
Today, photoautotrophs continue to maintain the balance of oxygen and carbon dioxide in the atmosphere. Through photosynthesis, they absorb carbon dioxide—a greenhouse gas—helping to mitigate climate change and release oxygen, which is essential for the respiration of most life forms, including humans. The oxygen produced by autotrophs is also crucial for the formation of the ozone layer, which protects life on Earth by filtering out harmful ultraviolet radiation from the sun.
What are the different sub-types of chemoautotrophs, and how do they obtain energy?
Chemoautotrophs are a diverse group of organisms that obtain energy by oxidizing inorganic compounds. They play a crucial role in nutrient cycling in ecosystems, particularly in environments where sunlight is absent. There are several sub-types of chemoautotrophs, each specialized in oxidizing different inorganic compounds:
- Nitrifying Bacteria:
- These bacteria oxidize ammonia (NH₃) to nitrite (NO₂⁻) and further oxidize nitrite to nitrate (NO₃⁻). Examples include Nitrosomonas and Nitrobacter. These bacteria are essential for the nitrogen cycle, converting ammonia into a form of nitrogen that plants can absorb and use.
- Sulfur Bacteria:
- Sulfur-oxidizing bacteria oxidize hydrogen sulfide (H₂S) to elemental sulfur (S) or sulfuric acid (H₂SO₄). Examples include Beggiatoa, Thiobacillus, and Thiooxidans. These bacteria are often found in sulfur-rich environments like hot springs and deep-sea hydrothermal vents. They play a vital role in the sulfur cycle by converting sulfur compounds into forms that can be used by other organisms.
- Iron Bacteria:
- Iron-oxidizing bacteria obtain energy by oxidizing ferrous iron (Fe²⁺) to ferric iron (Fe³⁺). An example is Ferrobacillus. These bacteria are typically found in iron-rich environments, such as wetlands and acidic mine drainage. They contribute to the iron cycle and play a role in the formation of iron deposits.
- Hydrogen Bacteria:
- These bacteria oxidize hydrogen gas (H₂) to produce energy, converting it into water (H₂O). An example is Hydrogenomonas. Hydrogen bacteria are found in environments where hydrogen is available, such as in soil, sediments, and the intestines of animals.
- Methane Bacteria:
- Methanotrophs or methane-oxidizing bacteria oxidize methane (CH₄) to carbon dioxide (CO₂). An example is Methanomonas. These bacteria are crucial in regulating methane levels in the environment, particularly in wetlands, where methane production is high.
Each of these sub-types of chemoautotrophs has adapted to specific ecological niches, playing a crucial role in recycling essential nutrients and maintaining the balance of chemical elements in ecosystems. Their ability to derive energy from inorganic compounds allows them to thrive in extreme environments where other forms of life cannot survive.
What is the ecological significance of autotrophic organisms in ecosystems?
Autotrophic organisms, primarily photoautotrophs, and chemoautotrophs, are of immense ecological significance as they serve as the foundation of the food chain in nearly all ecosystems. Their role as primary producers means they convert inorganic substances into organic matter that can be consumed by other organisms, known as heterotrophs.
- Energy Source for Heterotrophs:
- Autotrophs provide the primary source of energy for herbivores, omnivores, and carnivores in the ecosystem. For instance, plants (photoautotrophs) produce glucose through photosynthesis, which is consumed by herbivores such as deer. These herbivores, in turn, are prey for carnivores such as wolves. Without autotrophs, the energy flow in ecosystems would be disrupted, leading to the collapse of food webs.
- Oxygen Production:
- Photoautotrophs, such as green plants, algae, and cyanobacteria, produce oxygen as a byproduct of photosynthesis. This oxygen is essential for the respiration of aerobic organisms, including humans. The continuous production of oxygen by autotrophs is crucial for maintaining the balance of gases in the atmosphere and supporting life on Earth.
- Nutrient Cycling:
- Chemoautotrophs play a significant role in the cycling of essential nutrients such as nitrogen, sulfur, and iron. For example, nitrifying bacteria convert ammonia into nitrate, a form of nitrogen that plants can absorb. Sulfur bacteria oxidize hydrogen sulfide into sulfur compounds, which are essential for various biological processes. These nutrient cycles are vital for maintaining soil fertility and supporting plant growth.
- Climate Regulation:
- Autotrophs, particularly forests and oceanic phytoplankton, act as carbon sinks by absorbing carbon dioxide during photosynthesis. This helps mitigate the effects of climate change by reducing the concentration of greenhouse gases in the atmosphere. Forests, often referred to as the “lungs of the Earth,” play a significant role in sequestering carbon and regulating the global climate.
- Support of Extreme Ecosystems:
- Chemoautotrophs are essential for supporting life in extreme environments, such as deep-sea hydrothermal vents, where sunlight is absent. These organisms convert inorganic compounds into organic matter, providing a food source for unique communities of organisms, including giant tube worms and clams, that have adapted to these harsh conditions.
The ecological significance of autotrophic organisms cannot be overstated, as they are fundamental to the survival of life on Earth. They ensure the flow of energy, the cycling of nutrients, the maintenance of atmospheric balance, and the support of diverse ecosystems, both in sunlight-rich and extreme environments.
How does the autotrophic mode of nutrition differ between terrestrial and aquatic ecosystems?
The autotrophic mode of nutrition functions differently in terrestrial and aquatic ecosystems due to variations in environmental conditions, such as light availability, nutrient concentration, and the types of autotrophs present.
- Terrestrial Ecosystems:
- In terrestrial ecosystems, photoautotrophs such as green plants, mosses, and some algae dominate. These organisms are adapted to capture sunlight and perform photosynthesis efficiently in environments ranging from forests to grasslands and deserts. Terrestrial plants often have specialized structures like roots to absorb water and nutrients from the soil, leaves for capturing sunlight, and stomata for gas exchange. The availability of light is generally higher in terrestrial ecosystems, although it can vary with the density of vegetation and geographical location.
- Terrestrial ecosystems also include diverse plant species adapted to various climatic conditions. For example, cacti in deserts have evolved to conserve water and perform CAM photosynthesis, a specialized form of photosynthesis that minimizes water loss. Trees in tropical rainforests have broad leaves to capture maximum sunlight in the dense canopy.
- Aquatic Ecosystems:
- In aquatic ecosystems, photoautotrophs include phytoplankton, algae, and some aquatic plants like water lilies. Light availability decreases with depth, influencing the types of autotrophs that can thrive in different layers of the water column. Phytoplankton, which are microscopic photoautotrophs, are the primary producers in aquatic ecosystems and are found mostly in the upper layers of water where sunlight penetrates. These organisms form the base of the aquatic food web, supporting a wide range of marine and freshwater life.
- Aquatic ecosystems also support chemoautotrophs in environments where sunlight is absent, such as deep-sea hydrothermal vents. These organisms rely on chemical energy from the oxidation of inorganic compounds, such as hydrogen sulfide, to produce organic matter. Chemoautotrophs in these environments are crucial for sustaining life in the absence of sunlight, providing energy for unique communities of organisms.
The differences in light availability, nutrient concentration, and types of autotrophs between terrestrial and aquatic ecosystems lead to distinct adaptations in the autotrophic mode of nutrition. Terrestrial autotrophs are adapted to capture and use sunlight efficiently on land, while aquatic autotrophs have evolved to survive in various water depths and rely on both light and chemical energy sources.
What are the challenges faced by autotrophs in extreme environments, and how do they adapt?
Autotrophs living in extreme environments face numerous challenges, including limited availability of light, extreme temperatures, high pressure, and the scarcity of nutrients. However, many autotrophs have evolved unique adaptations to survive and thrive under these harsh conditions.
- Limited Light Availability:
- In environments such as deep-sea regions, where sunlight does not penetrate, chemoautotrophs have evolved to use chemical energy instead of light. These organisms oxidize inorganic compounds like hydrogen sulfide, methane, and iron to produce organic matter. Cyanobacteria found in the deep ocean have adapted to absorb faint light at extreme depths and can perform photosynthesis in low-light conditions.
- Extreme Temperatures:
- Autotrophs in hot environments, such as geothermal springs and hydrothermal vents, have adapted to high temperatures. Thermophilic bacteria and archaea, which are chemoautotrophs, thrive in these conditions by possessing heat-resistant enzymes that allow them to carry out metabolic processes at temperatures that would denature proteins in other organisms. Similarly, autotrophs in cold environments, such as polar regions, have developed mechanisms to function at low temperatures, including antifreeze proteins that prevent ice formation within cells.
- High Pressure:
- Autotrophs living in deep-sea environments face the challenge of high pressure, which can affect cellular processes. Many deep-sea chemoautotrophs have evolved specialized cell membranes and enzymes that remain functional under intense pressure. These adaptations enable them to maintain cellular integrity and continue metabolic activities despite the extreme conditions.
- Nutrient Scarcity:
- In nutrient-poor environments, autotrophs have developed strategies to maximize nutrient uptake and utilization. For example, some cyanobacteria can fix nitrogen from the atmosphere, allowing them to survive in environments where nitrogen is limited. Additionally, plants in nutrient-poor soils, such as pitcher plants and Venus flytraps, have evolved to obtain nutrients from other sources, such as capturing and digesting insects.
- Adaptation to High Salinity:
- Halophilic (salt-loving) autotrophs, such as certain types of algae and bacteria, have adapted to survive in high-salinity environments like salt flats and salt marshes. These organisms maintain osmotic balance by producing compatible solutes or by accumulating salts within their cells to prevent dehydration.
Through these adaptations, autotrophs have become remarkably resilient and capable of colonizing some of the most extreme environments on Earth. Their ability to harness energy from both light and inorganic chemicals, coupled with specialized adaptations, allows them to sustain ecosystems in places where most life forms cannot survive.
How do autotrophic and heterotrophic nutrition interact in ecosystems?
Autotrophic and Heterotrophic nutrition represent two fundamental modes of nutrient acquisition in ecosystems, and their interaction is crucial for maintaining ecological balance and energy flow. The relationship between autotrophs and heterotrophs can be summarized as follows:
- Energy Flow:
- Autotrophs, such as plants, algae, and certain bacteria, produce organic compounds through photosynthesis or chemosynthesis. These organic compounds, primarily carbohydrates, serve as the primary source of energy for heterotrophs. Heterotrophs include herbivores, carnivores, omnivores, and decomposers, all of which rely on consuming other organisms for their nutrition. Herbivores feed directly on autotrophs, while carnivores and omnivores consume other heterotrophs. The flow of energy from autotrophs to heterotrophs drives the structure and dynamics of ecosystems.
- Nutrient Cycling:
- The interaction between autotrophs and heterotrophs is essential for nutrient cycling. Autotrophs convert inorganic substances into organic matter, which heterotrophs use for growth and energy. When heterotrophs die, their bodies are decomposed by decomposers (such as bacteria and fungi), which break down organic matter into inorganic nutrients. These nutrients are then recycled back into the environment, where they can be taken up by autotrophs, completing the nutrient cycle. This process ensures the continuous availability of essential nutrients for all organisms in the ecosystem.
- Mutualistic Relationships:
- In many ecosystems, autotrophs and heterotrophs engage in mutualistic relationships that benefit both parties. For example, in mycorrhizal associations, fungi form symbiotic relationships with plant roots, enhancing the plant’s nutrient and water uptake in exchange for organic compounds produced by the plant through photosynthesis. Similarly, nitrogen-fixing bacteria in the roots of certain plants (like legumes) convert atmospheric nitrogen into a form that plants can use, while the plants provide carbohydrates to the bacteria.
- Ecosystem Productivity:
- The productivity of an ecosystem, or the amount of biomass produced, is largely determined by the activity of autotrophs. High primary productivity by autotrophs leads to greater energy availability for heterotrophs, supporting diverse and abundant communities. Conversely, ecosystems with low autotrophic productivity may have limited resources for heterotrophs, resulting in lower species diversity and abundance.
- Impact on Climate:
- Autotrophs play a role in climate regulation by absorbing carbon dioxide during photosynthesis, which affects the greenhouse gas levels in the atmosphere. Heterotrophs, through respiration, release carbon dioxide as a byproduct of energy metabolism. The balance between autotrophic carbon sequestration and heterotrophic respiration influences atmospheric carbon dioxide levels and climate change. Autotrophs help mitigate climate change by sequestering carbon, while heterotrophs contribute to carbon release.
The interaction between autotrophic and heterotrophic nutrition forms the basis of ecological dynamics and energy transfer in ecosystems. Autotrophs provide the essential organic matter and energy required by heterotrophs, while heterotrophs contribute to nutrient recycling and energy flow, ensuring the sustainability of ecosystems.
Read More Articles
- Ribosomes: The Cellular Machinery For Protein Synthesis
- The Five Kingdom Classification With Perfect Examples
- Eubacteria: Structure, Characteristics, Classification, And Types
- Archaebacteria: A Glimpse Into Ancient Life Forms
- Kingdom Protista: A Diverse Group Of Single-Celled Eukaryotes
- The Diversity And Importance Of Bacteria: Archaebacteria And Eubacteria
- Kingdom Monera: The World Of Bacteria