Slime Moulds are fascinating organisms that blur the lines between fungi, protozoa, and other eukaryotes. Once classified under fungi, they are now placed in the kingdom Protista due to their unique characteristics. These organisms exhibit a remarkable ability to transition between unicellular and multicellular states, depending on environmental conditions. Found in diverse habitats such as soil, decaying wood, and forest floors, slime moulds play a crucial role in nutrient cycling by decomposing organic matter. Their unique life cycle, involving both amoeboid and plasmodial stages, makes them an intriguing subject of study in biology.
In this article, we have gone through detailed information starting from the base knowledge of Biological Classification Systems to advanced detailed information about Slime Moulds.
Table of Contents
Introduction to Biological Classification
All living organisms on Earth are systematically grouped based on their similarities, dissimilarities, and phylogenetic relationships. This systematic arrangement of organisms into various groups and subgroups is known as biological classification. The importance of classification in biology cannot be overstated, as it aids in identifying and categorizing new organisms, provides insights into the evolutionary relationships among different species, and allows scientists to study representative organisms from each group to infer characteristics about the group as a whole.
By categorizing organisms into well-defined groups, biological classification helps simplify the complex diversity of life on Earth, making it easier to study and understand the various forms of life. Additionally, it facilitates the identification and study of new species, promoting a better understanding of the interrelationships among various organisms.
Historical Development of Classification Systems
Over the centuries, various systems of classification have been proposed, each with its own methodology and criteria. Understanding the evolution of these systems is essential to appreciate the modern classification approaches.
Artificial System of Classification
One of the earliest systems of classification was the Artificial System of Classification, also known as Aristotle’s Classification. This system, proposed by the Greek philosopher Aristotle, was based on a few superficial morphological characteristics, such as the presence or absence of red blood, the method of reproduction, and habitat. Aristotle divided living organisms into two broad groups: Plants and Animals. This system, though pioneering, was limited by its simplicity and the fact that it did not consider the evolutionary relationships between organisms. For example, this system classified organisms based on criteria such as whether they were flowering or non-flowering, or whether they were vertebrates or invertebrates.
Natural System of Classification
As scientific knowledge advanced, a more sophisticated approach was needed. The Natural System of Classification, also known as the Bentham and Hooker Classification, was developed in the 19th century by British botanists George Bentham and Joseph Dalton Hooker. This system was based on a comparative study of a larger number of characteristics, including morphological, anatomical, and reproductive traits. The natural system aimed to group organisms according to their natural relationships, reflecting their evolutionary history. This system marked a significant improvement over the artificial system, as it provided a more comprehensive and accurate representation of the diversity of life.
Phylogenetic System of Classification
With the advent of evolutionary theory, the need for a classification system that reflected the evolutionary relationships among organisms became apparent. The Phylogenetic System of Classification was developed, which grouped organisms based on their evolutionary history. This system, also known as the Engler and Prantl Classification or Hutchinson Classification, categorized organisms according to their evolutionary progression from primitive to advanced forms. This approach has become the foundation of modern biological classification, as it provides insights into the evolutionary processes that have shaped the diversity of life on Earth.
The Kingdom System of Classification
Building on the foundations laid by previous systems, the Kingdom System of Classification was developed, which categorizes all living organisms into distinct kingdoms. This system has evolved, leading to the development of various models, including the two-kingdom, three-kingdom, and five-kingdom classifications.
Two Kingdom Classification
The Two Kingdom Classification was the earliest attempt to group all living organisms into distinct categories. Proposed by Carl Linnaeus, known as the “Father of Taxonomy,” this system divided organisms into two kingdoms: Plantae and Animalia. The primary criterion for this classification was the presence or absence of a cell wall. Plants, which have cell walls, were placed in the kingdom Plantae, while animals, which lack cell walls, were placed in the kingdom Animalia.
However, this system had several limitations. It did not account for the differences between prokaryotes (organisms without a true nucleus) and eukaryotes (organisms with a true nucleus), or between unicellular and multicellular organisms. Additionally, it did not distinguish between autotrophic (self-feeding) and heterotrophic (feeding on others) organisms, leading to the classification of vastly different organisms into the same kingdom.
Three Kingdom Classification
To address the shortcomings of the two-kingdom system, the Three Kingdom Classification was proposed by the German biologist Ernst Haeckel. Haeckel introduced a new kingdom, Protista, to accommodate unicellular organisms that did not fit neatly into the kingdoms Plantae or Animalia. This system grouped all unicellular eukaryotes, including algae, protozoa, and fungi, under the kingdom Protista.
Haeckel’s three-kingdom system represented a significant advancement in biological classification, as it recognized the distinct nature of unicellular organisms. However, it still had limitations, particularly in its treatment of fungi and prokaryotes, which were not adequately distinguished from other organisms.
Five Kingdom Classification
The Five Kingdom Classification system, proposed by the American ecologist Robert H. Whittaker in 1969, represented a major breakthrough in biological classification. Whittaker’s system divided all living organisms into five distinct kingdoms: Monera, Protista, Fungi, Plantae, and Animalia. This system was based on several criteria, including cellular structure (prokaryotic or eukaryotic), the complexity of organization (unicellular or multicellular), mode of nutrition (autotrophic, heterotrophic, or saprophytic), mode of reproduction (asexual or sexual), phylogenetic relationships, and ecological roles.
- Kingdom Monera: This kingdom includes all prokaryotic organisms, such as bacteria and cyanobacteria (blue-green algae). These organisms are unicellular and lack a true nucleus.
- Kingdom Protista: This kingdom comprises unicellular and colonial eukaryotic organisms, such as protozoa, algae, and slime moulds. These organisms have a true nucleus and membrane-bound organelles.
- Kingdom Fungi: This kingdom includes eukaryotic, multicellular organisms that are primarily saprophytic, feeding on dead organic matter. Fungi have cell walls made of chitin and reproduce by spores.
- Kingdom Plantae: This kingdom consists of multicellular, autotrophic organisms that perform photosynthesis. Plants have cell walls made of cellulose and reproduce both sexually and asexually.
- Kingdom Animalia: This kingdom includes multicellular, heterotrophic organisms that lack cell walls and are capable of movement. Animals reproduce sexually and have specialized tissues and organs.
Whittaker’s five-kingdom system provided a more accurate and comprehensive framework for classifying the diversity of life on Earth. It recognized the fundamental differences between prokaryotes and eukaryotes, unicellular and multicellular organisms, and autotrophic and heterotrophic organisms.
Kingdom Protista: A Diverse Group of Eukaryotes
The Kingdom Protista is one of the most diverse groups of organisms, comprising a wide range of unicellular and colonial eukaryotic organisms. Protists are primarily found in aquatic ecosystems, where they form an essential component of the plankton community. They play a vital role in the ecosystem, serving as primary producers, decomposers, and predators.
General Characteristics of Protists
- Eukaryotic Cell Structure: Protists have a well-defined nucleus enclosed by a nuclear membrane, as well as membrane-bound organelles such as mitochondria, chloroplasts, and endoplasmic reticulum. This distinguishes them from prokaryotes, which lack a true nucleus and membrane-bound organelles.
- Diverse Modes of Nutrition: Protists exhibit a wide range of nutritional modes, including autotrophy (photosynthesis), heterotrophy (ingestion of other organisms), saprotrophy (decomposition of organic matter), and parasitism (feeding on a host organism). Some protists, such as Euglena, are mixotrophic, meaning they can switch between autotrophy and heterotrophy depending on environmental conditions.
- Motility: Many protists are capable of movement, using specialized structures such as cilia (short, hair-like projections), flagella (long, whip-like projections), or pseudopodia (temporary extensions of the cell membrane). For example, the Paramecium uses cilia to move and feed, while the Amoeba moves by extending and retracting its pseudopodia.
- Reproduction: Protists reproduce both sexually and asexually. Asexual reproduction typically occurs through binary fission, where the cell divides into two identical daughter cells. Sexual reproduction involves the fusion of gametes, resulting in genetic recombination and the formation of a zygote.
Subgroups of Protists
Protists are divided into several major groups based on their morphology, mode of nutrition, and ecological role. These groups include Chrysophytes, Dinoflagellates, Euglenoids, Protozoans, and Slime Moulds.
- Chrysophytes: This group includes golden algae and diatoms, which are primarily photosynthetic and form an essential part of the aquatic food chain. Diatoms have unique cell walls made of silica, which form intricate and beautiful patterns.
- Dinoflagellates: These are mostly marine, photosynthetic protists that have two flagella, one of which encircles the cell like a belt, while the other extends from one end. Some dinoflagellates, such as Gonyaulax, are responsible for harmful algal blooms known as “red tides,” which can produce toxins that affect marine life and humans.
- Euglenoids: Euglen
oids, such as Euglena, are mixotrophic protists that can perform photosynthesis in the presence of light and feed heterotrophically in the absence of light. They have a unique cell structure, with a pellicle (a proteinaceous layer) instead of a cell wall, allowing for flexibility and movement.
- Protozoans: Protozoans are primarily heterotrophic, unicellular protists that are often classified based on their mode of locomotion. For example, Amoeba moves using pseudopodia, Paramecium uses cilia, and Trypanosoma uses flagella.
- Slime Moulds: Slime moulds are fascinating organisms that exhibit characteristics of both protozoa and fungi. They are primarily saprophytic, feeding on decaying organic matter, and can exist as single-celled organisms or form large, multicellular structures under certain conditions.
Slime Moulds: Unique and Versatile Organisms
Slime moulds are an intriguing group of organisms that were previously classified under the kingdom Fungi due to their spore-producing fruiting bodies and saprophytic lifestyle. However, they have since been reclassified under the kingdom Protista, as they share characteristics with both fungi and protozoa.
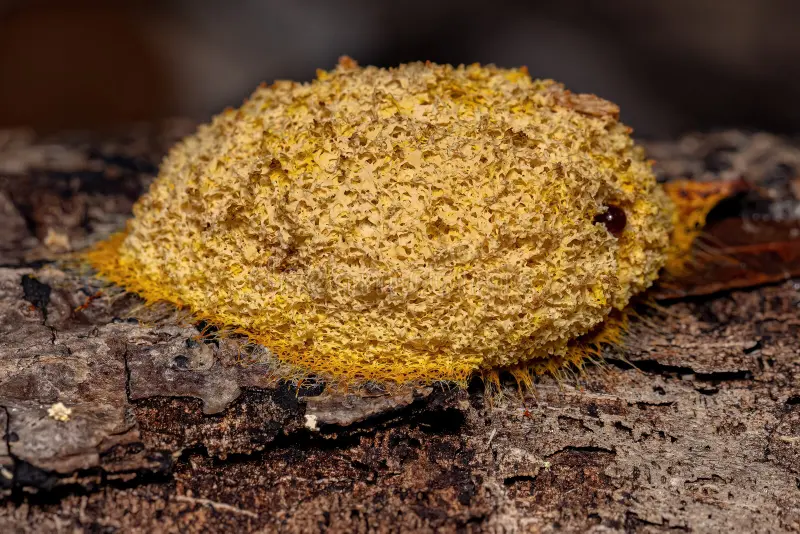
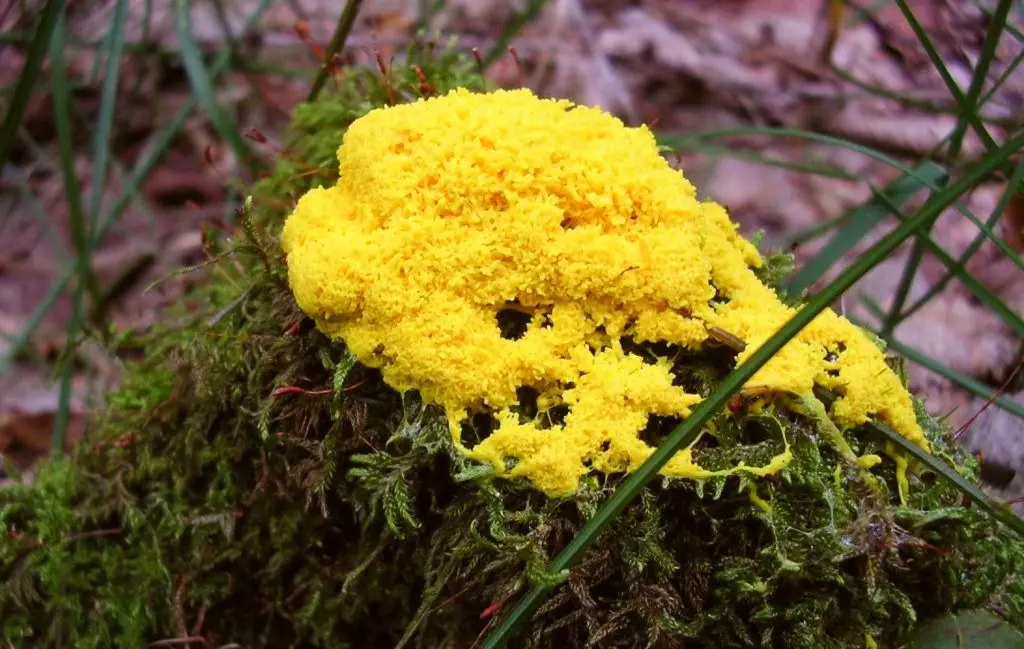
Morphology and Habitat of Slime Moulds
Slime moulds are named for their gelatinous or jelly-like appearance, which is most evident in the macroscopic stage of their life cycle. These organisms vary greatly in size, from a few centimetres to several square meters, and are typically found in moist, decaying environments, such as forest floors, leaf litter, and rotting wood.
Slime moulds are saprophytic, meaning they feed on dead and decaying organic matter, such as bacteria, fungi, and other microorganisms. This saprophytic lifestyle plays a crucial role in nutrient cycling and decomposition in ecosystems.
Classification of Slime Moulds
In modern taxonomy, true slime moulds fall under the phylum Mycetozoa within the kingdom Protista. They are further classified into several different classes based on their morphology, life cycle, and ecological role.
- Dictyostelium: Cellular slime moulds related to plasmodial slime moulds. They do not form large coenocytes and remain individual cells throughout most of their life cycle. Dictyostelium species are model organisms for studying cell differentiation, signalling, and development.
- Myxomycetes: Also known as plasmodial slime moulds, Myxomycetes are multinucleated plasmodia that undergo multiple nuclear divisions without cytokinesis, resulting in a syncytial (multinucleated) structure. They are characterized by their plasmodial stage, during which they can grow to cover large areas.
- Acrasiomycota: These are cellular slime moulds with a lifestyle similar to Dictyostelium. However, their amoebae have eruptive pseudopodia, which they use to move and engulf food particles.
- Protostelea: Intermediate between Myxomycetes and Dictyostelium, these are minute, simple, and amoeboid slime moulds that exhibit characteristics of both cellular and plasmodial slime moulds.
- Labyrinthulomycetes: Also known as slime nets, these marine slime moulds form a network of tubes through which amoeboid cells without pseudopodia can travel freely. They play a role in the decomposition of organic matter in marine environments.
- Plasmodiophoromycetes: These are primarily parasitic slime moulds that infect plants. Notable examples include Plasmodiophora brassicae, which causes clubroot disease in cabbages and other members of the Brassicaceae family, and Spongospora subterranea, which causes powdery scab in potatoes.
- Fonticula: These are cellular slime moulds that form a multicellular fruiting body in a unique volcano shape. Fonticula species are rare and are primarily found in soil and leaf litter.
Reproduction in Slime Moulds
The reproduction of slime moulds is a fascinating and primitive process that involves both sexual and asexual stages. In plasmodial slime moulds, the plasmodium contains numerous nuclei, each with two sets of chromosomes (diploid). When the plasmodium is exposed to light, it undergoes a dramatic transformation, forming fruiting bodies known as sporangia.
Within the sporangia, the nuclei undergo meiosis, a process that reduces the chromosome number by half, resulting in haploid spores. These spores are then dispersed by wind or water and can survive for long periods under adverse conditions. When they land in a suitable, damp environment, the spores germinate into amoeboid or flagellated cells, depending on the species.
These amoeboid cells, also known as gametes, are capable of sexual reproduction. In many species, the gametes are isogamous, meaning they are of similar size and shape. When two compatible gametes meet, they fuse to form a diploid zygote, which then develops into a new plasmodium, restarting the life cycle.
The Life Cycle of Slime Moulds
The life cycle of slime moulds is highly complex and involves both single-celled and multicellular stages. Depending on environmental conditions, slime moulds can exist as solitary amoeboid cells or aggregate to form large, multicellular structures.
- Cellular Slime Moulds: Under favourable conditions, slime moulds such as Dictyostelium exist as individual, single-celled amoebae that feed on bacteria and other microorganisms. When food becomes scarce, these amoebae aggregate to form a multicellular structure known as a pseudoplasmodium or slug. The pseudoplasmodium migrates to a suitable location, where it forms a fruiting body that produces spores. These spores are dispersed by wind or water and germinate into new amoebae under favourable conditions.
- Plasmodial Slime Moulds: Plasmodial slime moulds, such as Physarum polycephalum, exist as a large, multinucleated cell known as a plasmodium. The plasmodium is capable of extensive growth, spreading across surfaces to engulf and digest food particles. When conditions become unfavourable, the plasmodium undergoes a transformation, forming sporangia that produce haploid spores through meiosis. These spores germinate into haploid cells that can fuse to form a new diploid plasmodium.
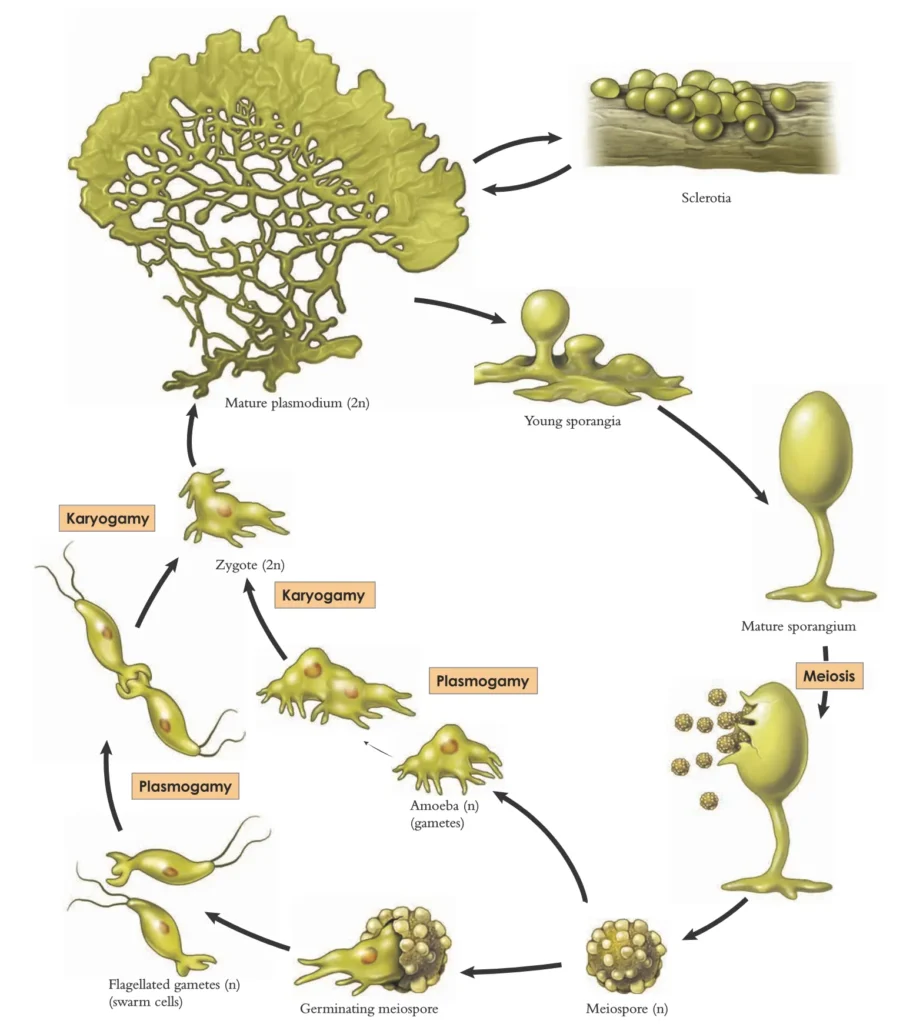
The life cycle of slime moulds is a complex interplay of growth, reproduction, and survival strategies. The cycle begins with the formation of sporangia on the drying plasmodium, which produces stalked fruiting bodies. Within these sporangia, meiosis occurs, resulting in the formation of haploid spores.
These spores are encased in a thick, resistant cell wall, allowing them to withstand harsh environmental conditions. Under favourable conditions, the spores germinate to produce haploid cells, which can act as gametes. Depending on the availability of moisture and other environmental factors, these haploid cells may develop into different forms, such as amoeboid or flagellated cells.
When two compatible haploid gametes fuse, they form a diploid zygote. This zygote undergoes multiple rounds of mitotic division without cytokinesis, resulting in the formation of a multinucleated plasmodium. This plasmodium continues to grow and spread, feeding on organic matter until environmental conditions trigger the formation of new sporangia, completing the life cycle.
General Characteristics of Slime Moulds
Slime moulds exhibit a range of unique characteristics that distinguish them from other organisms:
- Habitat: Slime moulds are typically found in moist, decaying environments, such as forest floors, leaf litter, rotting wood, and tree canopies. They thrive in dark, cold conditions and can be found creeping on debris, twigs, and leaves.
- Protoplast: In the vegetative phase, the protoplast (the living content of the cell) is not surrounded by a cell wall. This allows the plasmodium to change shape and move in response to environmental cues.
- Lack of Chlorophyll: Slime moulds do not contain chlorophyll and are therefore unable to perform photosynthesis. Instead, they are saprophytic, feeding on microorganisms such as bacteria, fungi, and yeasts, and decomposing dead organic matter.
- Parasitic Forms: Some slime moulds are parasitic, infecting the roots of plants, particularly those in the Brassicaceae family (such as cabbages). These parasitic slime moulds can cause significant agricultural damage, leading to diseases such as clubroot.
- Resilient Spores: The spores of slime moulds have a tough, cellulose-based cell wall that makes them resistant to adverse conditions. These spores can survive for many years, ensuring the persistence of the species through periods of environmental stress.
Economic and Ecological Importance of Slime Moulds
Slime moulds play a crucial role in ecosystems and have several economic and scientific applications:
- Nutrient Cycling: Slime moulds are important decomposers, breaking down dead organic matter and releasing nutrients back into the soil. This process is essential for maintaining soil fertility and supporting plant growth.
- Scientific Research: The plasmodium of slime moulds, particularly Physarum polycephalum, is widely used in scientific research. It is an excellent model for studying the structure and physiology of protoplasm, as well as cellular decision-making, motility, and network formation.
- Artistic Value: The vibrant colours and intricate patterns produced by some slime moulds have been used in artistic creations, particularly in the field of bio art.
- Agricultural Impact: While most slime moulds are beneficial as decomposers, some parasitic species can have negative impacts on agriculture. For example, Plasmodiophora brassicae causes clubroot disease, which can devastate crops in the Brassicaceae family, leading to significant economic losses.
Informative Table Related to Key Aspects of Slime Moulds
The table below summarizes key aspects of slime moulds, including their classification, characteristics, life cycle, habitat, and economic importance. This table provides a concise overview of the complex and fascinating nature of these unique organisms, highlighting their role in ecosystems and their significance in scientific research.
Aspect | Details |
---|---|
Kingdom | Protista |
Phylum | Mycetozoa |
Key Characteristics | Gelatinous appearance, saprophytic, exhibit both unicellular and multicellular stages |
Habitat | Moist, decaying environments such as forest floors, leaf litter, rotting wood, and tree canopies |
Life Cycle | Involves both single-celled (amoeboid) and multicellular (plasmodium) stages; sporangia produce spores that germinate into amoeboid cells |
Types of Slime Moulds | – Dictyostelium: Cellular slime moulds, remain as individual cells, form pseudoplasmodium under stress |
– Myxomycetes: Plasmodial slime moulds, multinucleated, form large coenocytic plasmodium | |
– Acrasiomycota: Cellular slime moulds with eruptive pseudopodia, similar to Dictyostelium | |
– Protostelea: Intermediate between Myxomycetes and Dictyostelium, minute and amoeboid | |
– Labyrinthulomycetes: Marine slime moulds, form slime nets for amoeboid cells to travel | |
– Plasmodiophoromycetes: Parasitic slime moulds, cause plant diseases like clubroot and powdery scab | |
– Fonticula: Cellular slime moulds, form multicellular fruiting bodies in a volcano shape | |
Reproduction | Involves sporangia forming haploid spores through meiosis; spores germinate into amoeboid cells that act as gametes; gametes fuse to form diploid plasmodium |
General Characteristics | – Lack of chlorophyll, feed on microorganisms (bacteria, fungi, yeasts) and decompose organic matter |
– Plasmodial stage is multinucleated, and spores have a cellulose-based cell wall resistant to adverse conditions | |
Ecological and Economic Importance | – Dictyostelium: Cellular slime moulds, remain as individual cells, and form pseudoplasmodium under stress |
– Used in scientific research, particularly Physarum polycephalum, for studying protoplasm and cellular processes | |
– Some species, like Plasmodiophora brassicae, are parasitic and cause significant agricultural damage, particularly in crops of the Brassicaceae family |
Conclusion
Slime moulds are a remarkable group of organisms that challenge traditional boundaries between kingdoms. With their unique life cycle, fascinating morphology, and ecological importance, slime moulds provide valuable insights into the diversity and complexity of life on Earth. Their ability to transition between unicellular and multicellular forms, their role in nutrient cycling, and their applications in scientific research make them a subject of enduring interest and significance in the study of biology.
Related Articles
- Euglenoid: The Unique Plant-Animal Hybrid
- Dinoflagellates: The Whirling Protists of the Oceans
- Chrysophytes: A Detailed Exploration Of Their Classification, Characteristics, & Significance
- Eubacteria: Structure, Characteristics, Classification, And Types
- Archaebacteria: A Glimpse Into Ancient Life Forms
- Kingdom Protista: A Diverse Group Of Single-Celled Eukaryotes
- The Diversity And Importance Of Bacteria: Archaebacteria And Eubacteria
- Kingdom Monera: The World Of Bacteria
- The Evolution Of Biological Classification Systems
- Understanding Taxonomy, Taxonomic Categories And Hierarchy
- The Diversity Of Life: Understanding Taxonomy, Classification, And Systematics
Frequently Asked Questions (FAQs) about Slime Moulds
What are slime moulds, and how are they classified?
Slime moulds are unique organisms that exhibit characteristics of both fungi and protozoa. They were previously classified under the kingdom Fungi due to their spore-producing fruiting bodies and saprophytic lifestyle. However, they have since been reclassified under the kingdom Protista, as they share more characteristics with other unicellular and colonial eukaryotic organisms within this kingdom. In modern taxonomy, true slime moulds fall under the phylum Mycetozoa.
What are the key characteristics of slime moulds?
Slime moulds are primarily saprophytic, meaning they feed on decaying organic matter, such as bacteria, fungi, and other microorganisms. They can exist as single-celled organisms or aggregate to form large, multicellular structures. Slime moulds have a gelatinous or jelly-like appearance, especially in their macroscopic stage. They lack chlorophyll and do not perform photosynthesis, relying instead on external sources of organic material for nutrition.
Where are slime moulds typically found?
Slime moulds are commonly found in moist and decaying environments, such as forest floors, leaf litter, rotting wood, and tree canopies. They thrive in dark, cold, and damp conditions, where they can easily access decaying organic material. Their presence is often associated with rich, nutrient-dense areas where decomposition is actively occurring.
How do slime moulds reproduce?
Slime moulds reproduce through a complex process involving both sexual and asexual stages. In the plasmodial stage, the plasmodium undergoes meiosis to produce haploid spores within fruiting bodies called sporangia. These spores are highly resistant to adverse conditions and can survive for long periods. When conditions are favourable, the spores germinate into an amoeboid or flagellated cells that can act as gametes. These gametes fuse to form a diploid zygote, which then develops into a new plasmodium.
What is the life cycle of slime moulds?
The life cycle of slime moulds involves alternating between single-celled and multicellular stages. The cycle begins with the formation of sporangia on the drying plasmodium, which produces stalked fruiting bodies containing haploid spores. These spores germinate into haploid cells under favourable conditions, which can act as gametes. The fusion of gametes results in the formation of a diploid zygote, which undergoes multiple rounds of mitotic division without cytokinesis to form a multinucleated plasmodium. This plasmodium continues to grow and spread, feeding on organic matter until it forms new sporangia, completing the life cycle.
What are the different types of slime moulds?
Slime moulds are classified into several different classes based on their morphology, life cycle, and ecological role:
- Dictyostelium: Cellular slime moulds that remain as individual cells and form a pseudoplasmodium under stress.
- Myxomycetes: Plasmodial slime moulds characterized by a large, multinucleated plasmodium that can cover extensive areas.
- Acrasiomycota: Cellular slime moulds with eruptive pseudopodia, similar in lifestyle to Dictyostelium.
- Protostelea: Minute, amoeboid slime moulds that are intermediate between Myxomycetes and Dictyostelium.
- Labyrinthulomycetes: Marine slime moulds that form slime nets through which amoeboid cells travel.
- Plasmodiophoromycetes: Parasitic slime moulds that infect plants, causing diseases like clubroot and powdery scab.
- Fonticula: Cellular slime moulds that form a volcano-shaped fruiting body.
How do slime moulds differ from fungi?
While slime moulds and fungi share some similarities, such as their saprophytic lifestyle and production of spores, they differ in several key ways. Slime moulds belong to the kingdom Protista, whereas fungi belong to the kingdom Fungi. Unlike fungi, slime moulds do not have a chitin-based cell wall and instead have a cellulose-based wall only in their spore stage. Additionally, slime moulds can exist as single-celled amoeboid organisms, while fungi are primarily multicellular organisms with a mycelial network.
What is the ecological importance of slime moulds?
Slime moulds play a crucial role in nutrient cycling and decomposition within ecosystems. As saprophytes, they break down dead organic matter and release nutrients back into the soil, supporting plant growth and maintaining soil fertility. Their ability to decompose complex organic materials helps maintain the balance of ecosystems by recycling essential elements like carbon, nitrogen, and phosphorus.
Are slime moulds harmful to plants or animals?
Most slime moulds are harmless to plants and animals, as they primarily feed on decaying organic matter. However, some parasitic slime moulds can cause significant damage to plants. For example, Plasmodiophora brassicae is a parasitic slime mould that causes clubroot disease in cabbages and other members of the Brassicaceae family. Another example is Spongospora subterranea, which causes powdery scab in potatoes.
How do slime moulds move and navigate their environment?
Slime moulds move using pseudopodia in their amoeboid stage, extending and retracting portions of their cell membrane to creep along surfaces. In the plasmodial stage, they exhibit a unique form of movement called protoplasmic streaming, where the cytoplasm flows rhythmically back and forth, propelling the organism forward. This movement allows slime moulds to explore their environment in search of food and avoid unfavourable conditions.
What is the scientific significance of studying slime moulds?
Slime moulds, particularly Physarum polycephalum, are valuable model organisms in scientific research. They are used to study a variety of biological processes, including cellular motility, differentiation, signalling, and network formation. Their ability to transition between unicellular and multicellular forms makes them ideal for studying developmental biology and evolutionary transitions. Additionally, their simple yet sophisticated behaviour in finding the most efficient paths through mazes has implications for research in artificial intelligence and biological computation.
How do slime moulds respond to environmental changes?
Slime moulds are highly responsive to changes in their environment. In response to favourable conditions, such as adequate moisture and temperature, they remain in their vegetative stage and continue to grow and feed. However, when conditions become unfavourable, such as drought or lack of food, slime moulds may enter a dormant stage by forming spores or aggregates into plasmodium to move to a better location. This adaptability allows them to survive in diverse and often challenging environments.
Can slime moulds be seen with the naked eye?
Yes, slime moulds can be seen with the naked eye, especially during their plasmodial stage when they form large, visible structures that can range from a few centimetres to several square meters in size. These structures are often brightly coloured, such as yellow, orange, or white, making them relatively easy to spot in their natural habitats. The fruiting bodies that produce spores are also macroscopic and can be seen without magnification.
What is isogamy, and how does it relate to slime moulds?
Isogamy refers to a form of sexual reproduction where the gametes (sex cells) are of similar size and shape. In slime moulds, isogamy occurs when haploid amoeboid cells (which act as gametes) are indistinguishable from one another in terms of size and morphology. When two compatible isogamous gametes meet, they fuse to form a diploid zygote, which then develops into a new plasmodium. This process of isogamy is typical in many slime moulds and contrasts with anisogamy, where gametes differ in size and form.
What are some examples of slime moulds and their significance?
Some notable examples of slime moulds include:
- Physarum polycephalum: A plasmodial slime mould widely used in scientific research due to its unique ability to solve complex mazes and form efficient networks, making it a model for studying biological computation and cellular behaviour.
- Dictyostelium discoideum: A cellular slime mould that serves as a key model organism for studying cell differentiation, chemotaxis, and developmental biology.
- Plasmodiophora brassicae: A parasitic slime mould that causes clubroot disease in cabbages and other cruciferous crops, leading to significant agricultural losses.
These examples highlight the diversity and importance of slime moulds in both natural ecosystems and scientific research.